Preface
The previous white paper focused on seasonal and daily changes to light quality (i.e., spectrum) and quantity (i.e., intensity and photoperiod), which significantly impact plant growth and development. While the impact of light quantity may appear more obvious, that of light quality is more abstract due to the nanoscopic nature of light. With light quantity, we can easily discern whether light is more intense and whether the day is longer or shorter. With light quality, we are considering the spectrum thus the decomposition of light into different colors, their wavelengths, and the relative amounts of each wavelength.
Light Quality
Light quality varies both seasonally and daily as weather conditions and the atmospheric path traveled by light impacts the absorption and scattering of different wavelengths, resulting in a discrepancy between pure sunlight and what reaches the plant canopy. Considering spectrum in terms of photon ratios has made it easier to study the impacts of light quality on plant growth and development, with the main ratios of interest being red to blue (R:B) and red to far-red (R:FR). Researchers are also beginning to study blue to green (B:G) and blue to far-red (B:FR), among others.
Understanding the morphological impacts of different photon ratios allows a grower to harness these ratios as a tool to guide plant development. For example, the ratio of R:FR light has been extensively studied due to its impacts on stem elongation, apical dominance, leaf expansion, photosynthetic efficiency, and flowering response in short-day and long-day plants. Controlling the R:FR ratio thus allows control over plant morphology architecture. With Sollum Technologies' dynamic LED lighting solution, growers can adjust the light spectrum at any point to achieve target ratios that result in desired morphological traits. To better understand the significance of this innovation, this white paper focuses on plant photoreceptors and the R:FR ratio.
Photoreceptors
Plants receive light as a source of energy for photosynthesis and information for metabolic processes, both of which are mediated by photoreceptors. Photoreceptors are a complex of proteins, and a pigment called a chromophore, the latter which selectively absorbs photons of specific wavelengths. Upon absorption of a photon, the chromophore triggers a change in the protein structure of the photoreceptors which in turn elicits an array of cellular responses in the plant.
Chlorophyll is perhaps the most well-known photosynthetic photoreceptor as its two main forms, a and b, are the most important photosynthetic pigments. Chlorophyll is responsible for the leaves’ green color due to the preferential absorption of red and blue light and the reflection of green light. The importance of chlorophyll is highlighted by the famous McCree Curve, which depicts a maximum photosynthetic efficiency induced by red and blue light. These maxima correlate with the absorption peaks of chlorophyll a (642 nm and 372 nm) and chlorophyll b (626 nm and 392 nm). However, photoreceptors absorb a range of wavelengths and non-chlorophyll photoreceptors contribute to photosynthesis to a lesser degree and/or indirectly. Research has also demonstrated that while far-red light falls outside chlorophyll's peak absorption range, it enhances the photosynthetic efficiency of red light through the Emerson effect. Red light is particularly efficient at driving photosynthesis because it is absorbed by both chlorophylls and phytochrome. Further, it is widely accepted that stomatal opening is partially regulated by blue-light photoreceptors, with water and carbon dioxide also strongly impacted stomatal functioning
Besides their important role in photosynthesis, photoreceptors strongly impact photomorphogenesis, phototropism, and photoperiodism.
Photomorphogenesis: light-mediated plant development, such as stem elongation, leaf thickening, etc. Strongly impacted by far-red and blue wavelengths.
Phototropism: growth of plants towards a light source. Strongly impacted by the direction of
the light.
As signaling molecules, photoreceptors communicate the ambient light conditions to the plant which then responds accordingly. For example, photoreceptors receiving low levels of red light communicate to the plant that it is being shaded. Depending on the species, plants react to low light levels through shade avoidance syndrome (SAS) which includes stem elongation, apical dominance and leaf expansion to grow past the obstruction and access more light.
Photoreceptors are present in all plant tissues: leaves, shoots and even roots. Moreover, the site of photoreceptor does not always correspond to the site of response, which means that the plant tissues intercepting light may be far away from the tissues or organs that react to it[i]. Not including chlorophylls and related pigments, there are five classes of photoreceptors for three categories of light. Phytochromes absorb primarily red (600- 700 nm) and far-red (700-750 nm) while cryptochromes, phototropins and zeitlupes absorb blue and UVA wavelengths. The photoreceptor UVR8 absorbs UVB light.
The importance of phytochromes will be further elaborated upon in subsequent sections. Cryptochromes play a role in plant shade avoidance, flowering induction and possibly temperature-dependent hypocotyl elongation and responses to changes in magnetic fields, as research studies suggest. Phototropins, which also respond to blue light, are responsible for light-mediated stomatal opening, chlorophyll translocation in plant tissues, and phototropism responses. Zeitlupes, the third class of photoreceptors that react to blue light, play an important role in maintaining the circadian rhythm, and can induce time-dependent flowering in some species. UVR8 photoreceptors are unique in that their activity is mediated not by a chromophore but by an aromatic molecule that absorbs UV-B light and triggers shade avoidance responses[ii].
The Phytochrome System: Short-and Long-Day Plants
Phytochromes were first discovered in the 1950s but research into their role in plant development is ongoing. Phytochrome preferentially absorbs red and far-red light and exists in two forms: Pr and Pfr. It is the relative concentration of Pr to Pfr that determines which physiological processes are triggered in the plant.
Phytochrome is a photoreversible molecule that switches between its active Pfr form and inactive Pr form based on the ratio of red to far-red light. The inactive form Pr quickly absorbs red light and transforms into the active Pfr form. This process is reversible, with the absorption of far-red light leading to the conversion of Pfr into Pr. The Pfr form also slowly reverts to the Inactive Pr form under dark conditions. Due to this reversibility, the phytochrome mechanism is often referred to as a molecular switch that clicks on in the presence of red light and off in the presence of far-red light. The on/off mechanism can have various implications in plant development including germination, chloroplast translocation and shade avoidance[iii].
During the day, high levels of red light in sunlight results in the conversion of most Pr into Pfr which signals to the plant that it is receiving significant light. During the nighttime without supplemental lighting, Pfr slowly converts back into Pr. This mechanism is how plants detect whether they are under long-day or short-day conditions, which is critical for the flowering of certain species (Figure 1).
In the case of short-day plants, long nights are necessary to complete the slow conversion of Pfr to Pr. The plant interprets the near-zero concentration of Pfr as a signal that the dark period is long enough, then begin its flowering process. In other words, the low levels of Pfr signals a lack of red light thus darkness. As such, Pfr inhibits flowering in short-day plants. Examples of short-day plants include poinsettia, Christmas cactus and chrysanthemum.
In long-day plants, Pfr encourages flowering as the short nighttime period results in incomplete conversion of Pfr to Pr, with the leftover Pr signalling to the plant that the nights are short enough to begin flowering[iv]. Examples of long-day plants include hibiscus, spinach, and potato.
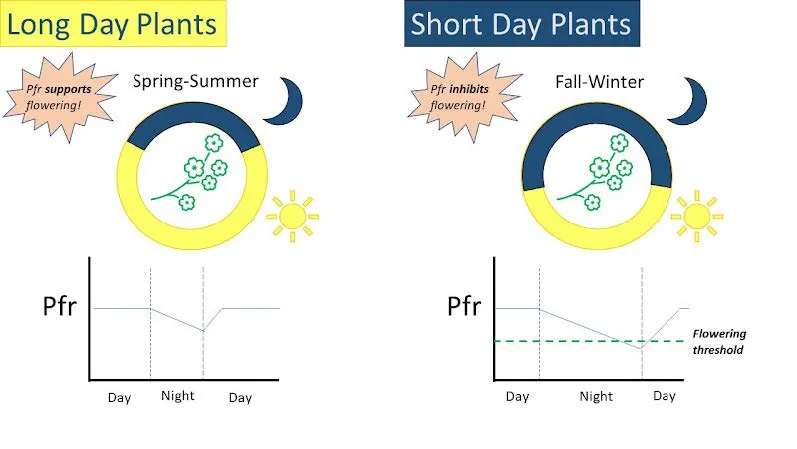
The Phytochrome System: Shade Avoidance
The phytochrome system also plays an important role in shade avoidance, through which plants employ various strategies to improve access to light. Plants are typically shaded due to overcrowding by other plants, long periods of overcast conditions and light obstruction by other objects. To mitigate shaded conditions, plants will commit energy to stem elongation (e.g., hypocotyl, internodes and petioles) and leaf expansion. A classic example of this is etiolation, through which the plant stretches to outgrow its light competitors and attain higher light levels[v].
The phytochrome system is one way by which plants initiate a shade avoidance response (Figure 2). Plants preferentially absorb red light, and their leaves reflect or transmit most far-red light[vi]. As a result, a shaded plant is exposed to a lower R:FR ratio than if it were in direct sunlight. Plants have several types of phytochrome photoreceptors, with Phytochrome B initiating the shade avoidance response. Like the flowering response, low Pfr concentrations are perceived by the plant as a low R:FR ratio indicating a lack of direct sunlight. This triggers the production of growth-promoting genes in the plants which then results in stem elongation and leaf expansion.

Manipulating the Red:Far-Red Ratio With Dynamic Lighting
Thanks to the reversibility of the phytochrome system, growers can cause different morphological changes in their crop by manipulating the ratios of red and far-red light, which consequently impacts the concentration of Pfr and Pr. For greenhouse ornamentals, managing the R:FR ratio allows growers to either accelerate or decelerate flowering, stretch plants and choose between the dominance of a single head or branching. In most cases, growers of ornamentals prefer to have a compact crop that is near flowering upon purchase. For bedding plants, compactness is essential although the plants can be a little further from flowering. Either way, consumers expect a certain level of stretch and nearness to flowering upon purchase. Managing the R:FR ratio is a powerful tool for meeting these expectations.
With the majority of greenhouse food crops being day-neutral, the benefit of controlling the R:FR ratio lies less in flowering response and more in plant architecture. For example, internode length has proven important in the production of greenhouse vine crops as it dictates how much space fruit has to develop. In pepper production, short internodes result in pepper stacking whereby developing peppers are closely stacked which limits their vertical development, producing a stocky pepper. Conversely, cucumber operations could benefit from shorter internodes as the crop's quick growth means the plant need to be lowered twice a week. Reducing internode length would result in a shorter crop thus less frequent lowering. An emerging use of far-red light is in the early stages of greenhouse strawberry development. As strawberry plants are somewhat compact, leaf overlap can limit light capture thus photosynthetic activity. By applying far-red during the first few weeks following transplants, growers can ensure that stems are sufficiently long and the leaves fall away from the crown, improving both light capture and airflow through the canopy.
Significant research is being conducted on the additional benefits of R:FR management such as the impacts on plant yield, time to harvest, leaf thickness and biomass accumulation. For example, Kalaitzoglou et al. (2019) investigated the effects of continuous low R:FR and end-of-day far-red treatments on greenhouse tomato plants[viii]. Rather than using the R:FR ratio as a metric, the study used the phytochrome stationary state (PSS), which is a more precise indicator of phytochrome status by accounting for phytochrome' sensitivity to other wavelengths. However, it is similar in principle to R:FR in that a low PSS indicates a low R:Fr ratio and vice versa. According to Kalaitzoglou et al. (2019), increasing the PSS above the solar PSS resulted in increased plant height but lower leaf area and plant dry mass. As such, lower PSS values increased the overall light absorption and photosynthetic rate by expanding the leaf area. This was positively correlated to greater fruit production, which the authors posit is due to the higher source strength generated by the larger leaves. Finally, the researchers reported that continuous application of far-red light during the day was more effective than end-of-day treatments, although the latter was still useful for situations where there is no far-red during the day. In these cases, end-of-day far-red treatments could help avoid undesirable impacts associated with the complete absence of far-red such as delayed flowering and reduced fruit production.
These results were supported by Ji et al. (2019), who reported an increase in total fruit dry mass when far-red light was added (thus decreasing the R:FR ratio). The researchers also reported that truss appearance was 11-14% faster in treatments with 30 or 50 μmol/m2/s of far-red[ix].
Similarly, research by Legendre and van Iersel (2021) demonstrated that supplemental far-red lighting enlarged leaf length and width of lettuce, which was associated to higher light capture and increased dry matter accumulation. The study also reported that adjusting far-red-light levels was more effective at building up plant biomass than light intensity[x].
With Sollum Technologies' fully dynamic LED lighting solution, growers have elongation, leaf expansion and time to harvest. Furthermore, Sollum Technologies' dynamic lighting system is a future-proof solution that allows growers to adapt their lighting strategy as our understanding of photoreceptors and the role of photon ratios evolves.
In the next white paper, we will further elaborate on what dynamic lighting is and how it is being used to push the boundaries of horticultural lighting.
About Sollum Technologies
Inspired by nature, Sollum Technologies was founded in 2015 to offer greenhouse growers the only smart LED lighting solution which dynamically recreates, perfects, and modulates the full spectrum of the Sun's natural light. The company is based in Montréal (Québec, Canada), where its design, development, and manufacturing activities are located. Sollum works closely with its clients and research partners namely, Agriculture and Agri-Food Canada's Harrow Research and Development Center, to create recipes that are adapted to the growth cycle of each crop, regardless of its native climate or the location of the greenhouse. Its SUN as a Service® cloud platform enables multi-zone light management so growers can implement several different recipes simultaneously in the same greenhouse. The platform also automatically adapts the lighting of each zone to the ambient light to match recipe targets. Sollum's award-winning lighting solution thereby provides unparalleled value in terms of energy savings, productivity, and superior produce quality through a flexible, adaptive, and easy-to-use application, with great respect for the environment. For more information, visit sollum.tech or our LinkedIn page.