Preface
In the last white paper, the concept of dynamic lighting was introduced and four key criteria that a truly dynamic solution needs to meet were identified:
- Output intensity that can be changed effortlessly;
- Light spectrum that can be modified and tailored endlessly;
- Programmable and reprogrammable lighting scenarios;
- Responsiveness to ambient light.
With these criteria in mind, some of the benefits of dynamic lighting were described - one of which was improved energy efficiency. In this white paper, the topic of energy efficiency will be discussed in greater detail by considering how a truly dynamic lighting solution can apply spectral compensation, in addition to intensity regulation, to preserve energy in a greenhouse.
Introduction
It makes environmental and economic sense for growers to invest in energy efficient lighting. Worldwide, greenhouses alone account for an estimated electricity equivalent of 244,640 million kilowatt hours in energy consumption (Katzin et al., 2021). Energy costs can constitute 50% of total greenhouse operational costs in some cases (Shen et al., 2018), and most of that proportion can be attributed to lighting (Watson et al., 2018). As indoor agriculture continues to play an increasingly important role in global food security, it is imperative that greenhouse lighting systems are optimized for energy efficiency.
Besides environmental and economic costs, energy availability is also a limiting factor for greenhouse operation and development. Recent growth in the number of greenhouse projects, the popularity of artificial lighting systems for greenhouses, and the desire for year-round production, have put significant strain on local energy grids. In Essex County, Ontario (Canada), North America's greenhouse capital, facility expansions are constrained and delayed due to electrical grid limitations. For existing greenhouses, energy supply is subject to interruptions during periods of peak demand - such as during colder periods (Posterity Group, 2019). Meanwhile, in the Netherlands - the greenhouse capital of the world - escalating fuel prices are causing entire greenhouses to either significantly scale back operations or shut down entirely (Quinn et al., 2021). In Québec (Canada), growers who implement ‚"photosynthetic lighting or space heating" are offered a reduced rate for the electricity consumed by these additional systems, however this special rate only applies during "authorized periods" that fall outside of peak demand times (e.g. winter mornings and afternoons); During "unauthorized periods" the price of additional electricity increases tenfold and growers must typically supplement their energy needs using a generator (Hydro- Québec, n.d.). Efficiency improvements are critical for creating cap space for new greenhouse operations and expansions, maintaining peak productivity during cold and dark periods, and ensuring that essential agricultural services can withstand fluctuations in energy prices.
It is no secret that light emitting diodes (LEDs) are the most energy efficient lighting option available to growers. Compared to conventional lighting options, LEDs can use up to 70% less energy (Singh et al., 2015). Compared to high pressure sodium fixtures (HPS) alone, LEDs are 60% more efficient at converting electricity to photosynthetically useful light (Katzin et al., 2021). Additionally, LEDs have dimming capabilities, meaning that their output intensity can be reduced without significantly impacting their spectral character. Intelligent LED systems, like Sollum Technologies’ AI-driven dynamic lighting solution - that are responsive to changes in ambient light intensity - can further reduce their energy consumption by dimming when sunlight is available (Elkins and Iersel, 2020). Because maintaining an appropriate and consistent daily light integral (DLI) is key to optimizing crop yield (Albright et al., 2000), increasing and decreasing output intensity in response to changes in ambient light not only leads to energy savings but increases greenhouse productivity overall.
While there is a wealth of available research to support LEDs for their energy efficiency, and basic dimming operations represent a common-sense solution for further energy savings, there has been minimal discussion of the role that spectral compensation can play in reducing electricity consumption. Spectrally dynamic lighting solutions have an edge over alternatives because, in addition to possessing all the benefits of other intelligent lighting systems, they can use dynamic spectral capabilities to optimize greenhouse production in terms of productivity and energy use. While the switch from HPS to LED can reduce energy consumption in a greenhouse by 40%, preliminary research suggests that switching from conventional LEDs to a dynamic smart LED system can increase energy savings by an additional 20%; the reasons for this substantial jump in efficiency will be explored in the following sections.
Spectral compensation
Spectral compensation is only made possible using truly dynamic variable spectrum lighting systems such as Sollum Technologies' smart LED solution. In the same way that dynamic lights can modulate their output intensity based on changes in ambient light, they can modulate their output spectra based on the ambient spectrum. The spectral makeup of sunlight varies based on weather and climate and the spectral needs of plants vary based on crop and growth stage. When spectral compensation is not available, growers can only attempt to accommodate the light quality needs of their crops by adjusting the intensity of their fixed spectrum supplemental lights.
For instance, if crops are lacking in one wavelength band but spectral compensation is not available, the overall intensity of the light will have to be raised, which increases the availability of all wavelengths in the light's spectrum. From an energy standpoint, meeting a crop's lighting needs using intensity control alone is inefficient because making a light brighter to fill gaps in spectra consumes unnecessary additional electricity. It also fails to consider how wavelength ratios impact plant photoreceptors and resulting growth patterns of response (Seguin, 2022). Using spectral compensation, wavelengths can be targeted specifically by adjusting a light's spectral output in real time without an unnecessary increase elsewhere in the spectrum.
A result of employing spectral compensation in combination with intensity modulation is that the total DLI of a crop can be reduced during periods of peak electrical grid demand without interrupting the productivity of the crop. If precision lighting systems that offered dynamic compensation were adopted by all greenhouses, pressure from the sector on utility providers could be greatly reduced and barriers to new projects and expansions could be removed.
In the following sections, the concept of spectral compensation in conjunction with intensity modulation will be elaborated upon using detailed examples.
Total dynamic real-time sunlight compensation can only be achieved using advanced variable spectrum capable lighting.
Compensating for weather
An earlier white paper titled "Seasonal Variation in Natural Light" discusses in detail how sunlight changes both seasonally and daily in terms of both intensity and spectra. To recap, the major factors that contribute for variation in natural light over the course of a day or a year are solar elevation angle - i.e., how high the sun is in the sky and the resulting angle of incident sunlight on the Earth's surface - and atmospheric composition which results in differential scattering of light by particles in the atmosphere (Seguin, 2022). Without spectral compensation, a smart lighting solution's only manner of response to a cloudy day, for instance, would be to increase lighting intensity - thus consuming more electricity overall. However, the relationship between weather and natural light is complex and cannot be addressed by considering brightness alone.
Researchers Chiang et al., (2019) conducted an in-depth study that tracked all the spectral changes of natural light at a mid-latitudinal location over the course of an entire year. Their findings highlight the significant impacts of atmospheric weather events on the natural light available to plants and how weather and climate factors interact to further complicate light patterns. In a general sense, from sunrise to sunset, the researchers observed that blue light (400 - 500 nm) will decrease as the day progresses and green (500 - 600 nm), red (600 - 700 nm) and far-red (700 - 780 nm) wavelengths will increase (figure 1.)
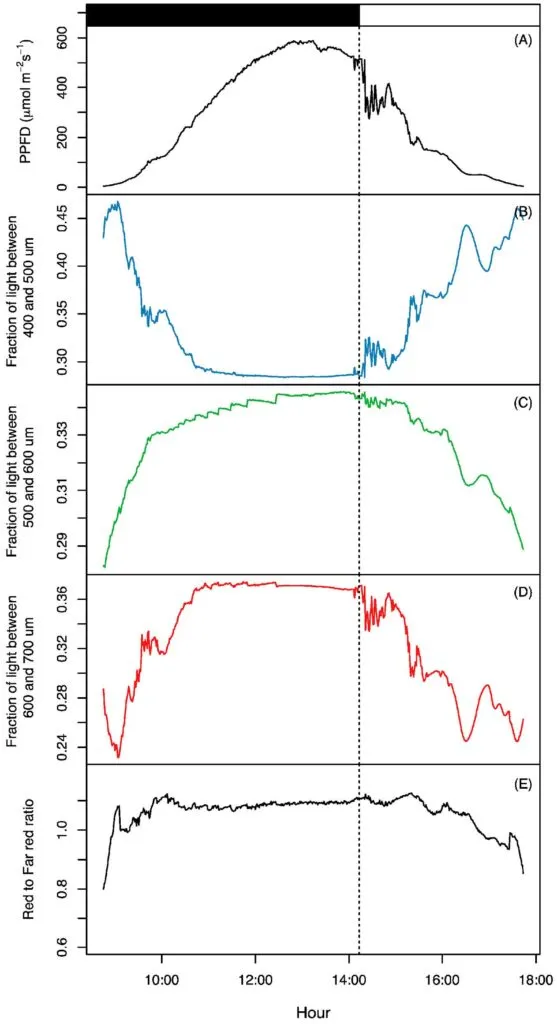
They found that the solar elevation angle and weather events together had compounding effects. For instance, at low solar elevation angles - i.e., during sunrise or sunset - the red to far-red light ratio maybe 0.8 on a cloudy day and 1.3 during clear sky conditions. At solar elevation angles lower than 20°, atmospheric weather conditions will strongly impact available red and blue wavelengths in the light spectra such that a significantly higher proportion of red light and a lower proportion of blue light will result. During mid-day - at higher solar elevation angles - the opposite trend was observed but to a lesser degree with the proportion of blue light increasing marginally with increasing cloud cover, and the proportion of red light decreasing marginally.
A truly dynamic lighting solution - like that of Sollum Technologies' - can address weather variation by adjusting light spectra as well as light intensity in immediate response to changes in ambient light. For other smart lighting systems, mimicking clear sky conditions on a cloudy day can only be attempted by increasing brightness - thus consuming additional electricity that may not be necessary. Precise sunlight compensation fine-tuning both the spectrum and intensity leads to energy savings.
The following graphic provides an example of dynamic compensation as accomplished by one of Sollum Technologies' smart LED grow light. In this example, the following light recipe is precisely and accurately maintained thanks to dynamic compensation: LED lights turn on early in the morning - before sunrise -ì and recreate the full sunlight spectrum including far-red (the grower may choose to include far-red for several reasons such as to promote shoot elongation during vegetative growth in crops). As the Sun rises, not only is more photosynthetically active radiation (PAR) available to the plants, but more far-red light is available as well, and the ratio of blue to red light decreases. Responding in real time, the intensity of the LED lights is reduced, far-red is deactivated and the fixture's blue:red wavelength ratio output is increased. As the Sun sets, the intensity of the LEDs rises, the spectrum shifts accordingly and far-red is reactivated. This is only one possible light recipe out of an infinite number that may be employed using Sollum Technologies' smart LED grow light solution.

Precise sunlight compensation fine-tuning both the spectrum and intensity components leads to energy savings.
Compensating for growth stages
In addition to addressing variation in terms of weather and climate, dynamic lighting and spectral compensation can also be used to accommodate varying needs of plants during different growth stages. Fine tuning artificial lights to precisely provide what a plant needs, when it needs it, will improve overall efficiency - i.e., the kWh/kg ratio. The more optimized a greenhouse facility is for the growth of the harvested crop, the less energy input is needed relative to the biomass of the crop yield.
Put another way, optimized greenhouse operations can output higher quality yields for the same amount of energy consumed by a less efficient facility. Specific lighting treatments can be used to trigger metabolic responses that improve plant anatomy, chemical composition, physiology, growth and reproduction - effectively providing a grower "more bang for their buck" energy-wise.
Even in the earliest stages of plant growth, differential application of spectra and intensity can result in significant overall energy and economic savings (Chang et al., 2014: Poulet et al., 2014). Poulet et al. (2014) found that the energy conversion rates of lettuce (the rate at which lettuce plants convert light energy to biomass), grown under LED lighting vary depending on growth stage.
During the lag phase of growth (the first 10-15 days past germination) they found that 0.35 g of biomass per kWh of light was produced; this ratio increased to 2.78 g/kWh during the exponential phase of growth (when growth is fastest). The difference in the amounts of electricity consumed by the growing operations during these phases (17 kWh during the lag phase versus 26.8 kWh during the exponential phase was much less pronounced (1:1.6 compared to 1:8). In other words, a significant amount of supplemental light did contribute to crop biomass during the lag phase of growth. Given their findings, these researchers emphasized that there is much room for lighting optimization during the early stages of plant growth to achieve significant energy savings.
For other crops - such as cucumbers and tomatoes - there are distinct vegetative and fruiting stages of growth before harvest that also benefit from differential light treatments. Blue light promotes vegetative growth- the development of shoots and leaves-while red light promotes the development of flowers and fruit (Xu et al., 2016). As discussed in the previous section, the ratio of red to blue wavelengths available in natural light can change depending on environmental factors. A dynamic lighting solution can account for changes in ambient light due to environmental factors in order to maintain a specific lighting recipe that considers the growth stages of a crop; this way, energy is conserved when wavelengths for a crop's distinct growth stage are sufficiently present in ambient light.
Finally, a dynamic lighting solution can also improve energy efficiency simply by improving the growth rate of crops. Chang et al., (2014) showed that when the output spectrum and intensity of supplementary lighting was precisely managed and purposefully regulated to meet plant needs, the growing period of lettuce could be significantly reduced, thus resulting in energy savings.
Specific lighting treatments can be used to trigger metabolic
responses improving plant anatomy, chemical composition,
physiology, growth, and reproduction.
Conclusion
Reducing energy consumption by improving greenhouse lighting efficiency plays a critical role in managing environmental and economic costs of the industry, making room for new facilities and expansion, and improving greenhouse resiliency as year-round indoor agriculture becomes increasingly necessary for ensuring food security. Beyond the already substantial energy efficiency associated with LED lights, preliminary research suggests that installing a dynamic lighting solution like that of Sollum Technologies' can reduce electricity consumption by an additional 20%. Innovation in intensity regulation and spectral compensation drives the added savings by intelligently filling gaps in available ambient light and adapting over the course of a growing cycle to perfectly meet a crop's changing needs.
In the next white paper another benefit of dynamic lighting will be discussed in detail by considering how innovation can be found in terms of variety and scale in greenhouse productions.
About Sollum Technologies
Inspired by nature, Sollum Technologies was founded in 2015 to offer greenhouse growers the only smart LED lighting solution which dynamically recreates, perfects, and modulates the full spectrum of the Sun's natural light. The company is based in Montréal (Québec, Canada), where its design, development, and manufacturing activities are located. Sollum works closely with its clients and research partners namely, Agriculture and Agri-Food Canada's Harrow Research and Development Center, to create recipes that are adapted to the growth cycle of each crop, regardless of its native climate or the location of the greenhouse. Its SUN as a Service® cloud platform enables multi-zone light management so growers can implement several different recipes simultaneously in the same greenhouse. The platform also automatically adapts the lighting of each zone to the ambient light to match recipe targets. Sollum's award-winning lighting solution thereby provides unparalleled value in terms of energy savings, productivity, and superior produce quality through a flexible, adaptive, and easy-to-use application, with great respect for the environment. For more information, visit sollum.tech or our LinkedIn page.